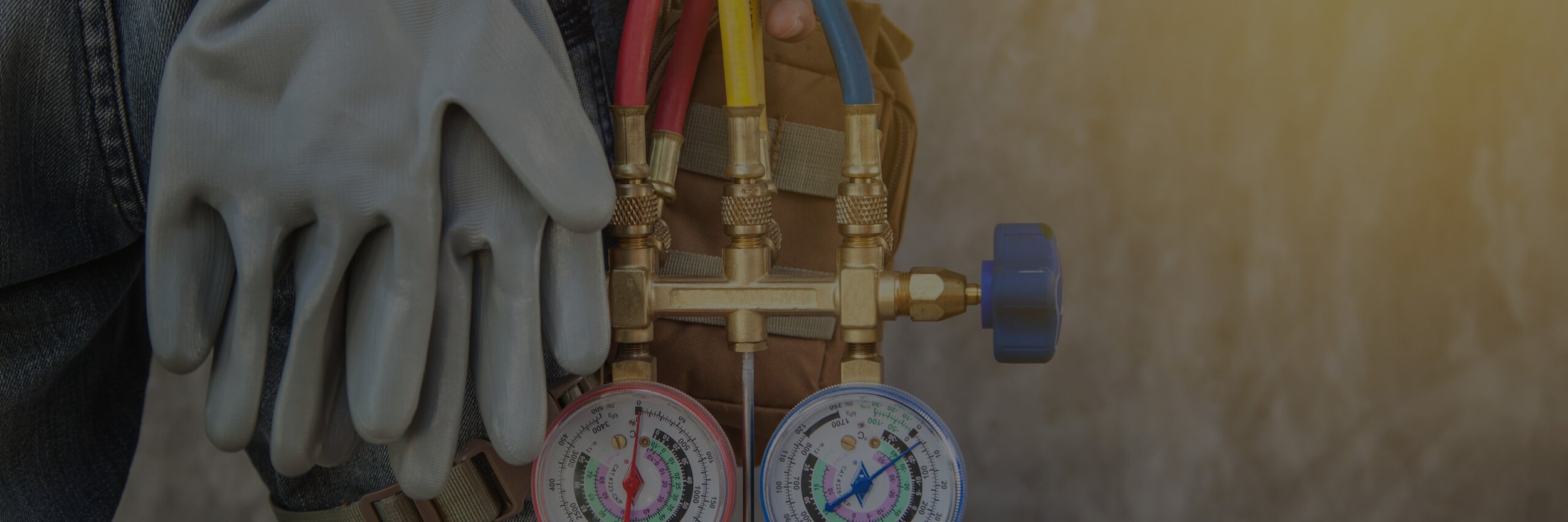
Building Green:
The Role of Thermodynamics in Sustainable HVAC Design
Thermodynamics of Refrigeration
Building Green: The Role of Thermodynamics in Sustainable HVAC Design
by Jonathan Kilburn
With the increasing global emphasis on sustainability and environmental preservation, the field of Heating, Ventilation, and Air Conditioning (HVAC) is experiencing a shift. The application of thermodynamics in HVAC design has been pivotal in transforming traditional methods into green and sustainable practices. Let’s look into the role of thermodynamics in creating energy-efficient HVAC systems and provide tangible examples of its real-world application.
Understanding Thermodynamics in HVAC
Thermodynamics, at its core, studies the movement and conversion of energy. In the context of HVAC, thermodynamics helps understand how energy is used, transferred, and conserved within a system.
First Law of Thermodynamics:
The principle of energy conservation plays a critical role in ensuring that HVAC systems are designed to use energy effectively without unnecessary waste. This principle ensures that HVAC systems, like the one implemented in the Willis Tower building design in Chicago, are designed to balance energy input with output, maintaining efficiency without unnecessary waste.
Second Law of Thermodynamics:
This law emphasizes that some energy is always lost as waste heat in any energy transfer. Recognizing and mitigating this loss is essential for sustainable design. The loss of energy as waste heat is recognized and mitigated, as seen in the Apple Park campus, where innovative designs limit energy loss to improve overall sustainability.
The Cornerstones of Sustainable HVAC Design
By applying thermodynamic principles, several key areas are addressed in sustainable HVAC design.
Energy Recovery:
Within HVAC systems, this refers to an energy system’s process of capturing and reusing waste heat or cooling that would otherwise be lost to the environment. This practice is essential for improving energy efficiency, reducing operational costs, and having a minimal environmental impact. Energy recovery systems, like heat recovery ventilators (HRVs) or energy recovery ventilators (ERVs), transfer energy from exhaust air to precondition fresh incoming air. In the winter, waste heat from inside the building is captured to warm the cold incoming air. Conversely, in the summer, the cooler exhaust air can precondition incoming hot air. This continuous exchange not only conserves energy but also helps maintain a comfortable indoor climate, emphasizing sustainability in modern HVAC design.
As a good case study, the Bullitt Center in Seattle uses a heat recovery system that significantly reduces heating costs, embodying the principle of recycling energy.
Renewable Energy Integration:
This involves incorporating renewable energy sources such as solar, wind, or geothermal energy to power heating, cooling, and ventilation functions to the HVAC systems. This approach significantly reduces dependence on fossil fuels, aligning HVAC operations with sustainability goals. For example, solar panels can be used to generate electricity for air conditioning, or geothermal energy can be tapped for both heating and cooling. The integration of these renewable sources requires thoughtful design and may include hybrid systems that use traditional energy sources as backups. By embracing renewable energy, HVAC systems can not only reduce greenhouse gas emissions but also lower operating costs, fostering a more responsible and environmentally conscious approach to temperature control and air quality management.
The Eden Project in the UK has a building system that taps into geothermal energy for climate control, aligning with a vision of harnessing renewable resources.
System Optimization:
In short, System Optimization is customizing and fine-tuning heating, cooling, and ventilation systems to meet a building's specific needs and requirements efficiently. It involves the strategic selection, design, and operation of equipment and controls to minimize energy consumption while maintaining optimal comfort levels, often done by a mechanical engineer but adapted by HVAC professionals. Techniques might include variable speed drives that adjust motor speeds according to demand, zone controls that allow different areas to be heated or cooled independently, or using sensors to adapt to external weather conditions. For example, ice storage systems that produce ice during off-peak hours for cooling during the day or incorporating smart thermostats that learn occupant behavior. System Optimization is vital for achieving both sustainability goals and operational savings, by reducing unnecessary energy use and promoting an overall more intelligent and responsive HVAC system.
One Bryant Park in New York, not just a commercial building, applies the concept of tailored design through ice storage and variable speed chillers, aligning energy use with demand.
Geothermal Heat Pumps:
A Geothermal Heat Pump (GHP is a highly efficient system that uses the Earth's constant underground temperature to provide heating and cooling. Unlike traditional systems that rely on outdoor air, GHPs harness the stable thermal energy found below the Earth's surface and maximizes energy performance. During winter, the system extracts heat from the ground and transfers it indoors, and in summer, it reverses the process, taking heat from inside the building and moving it into the ground. This creates a continuous, energy-efficient loop. The use of geothermal energy significantly reduces the reliance on fossil fuels and electrical consumption, resulting in lower greenhouse gas emissions and energy costs. By tapping into a renewable and abundant energy source, Geothermal Heat Pumps epitomize sustainable design and innovation within the HVAC industry. This is done with a wide range of refrigerants or glycol in order to create a separate loop.
Ball State University's massive geothermal system showcases how consistent underground temperatures can be harnessed for heating and cooling.
Passive Solar Design:
While similar to Renewable Energy Integration, Passive Solar Designs are an approach that leverages the sun's energy to naturally heat and cool a building without relying on mechanical systems. It requires careful planning in the design phase, considering factors such as building orientation, window placement, insulation, and materials that can absorb and store solar heat. During winter, large south-facing windows can capture sunlight and convert it into heat, stored in materials like concrete or brick. At night, this stored heat is released, maintaining a comfortable temperature. In summer, shades, overhangs, or other cooling strategies are used to block excessive heat. Passive Solar Design not only minimizes energy consumption and costs but also contributes to a building's sustainability by reducing dependence on conventional heating and cooling systems. It's an elegant integration of architecture and energy efficiency.
Wisconsin's Aldo Leopold Legacy Center effectively utilizes solar energy through proper orientation and insulation, a stellar example of energy-efficient design.
Variable Refrigerant Flow (VRF) Systems:
Tokyo's Mode Gakuen Cocoon Tower employs VRF technology, allowing individualized temperature control while conserving energy.
As the industry progresses, several emerging technologies are set to shape the future of HVAC
Building Information Modeling (BIM): BIM facilitates more accurate design and simulation, enabling energy-efficient planning. Many companies focus on this aspect heavily in industrial settings, but the same practice can be applied to residential use and design.
Smart Sensors and IoT Integration:
Smart Sensors and IoT (Internet of Things) Integration in HVAC systems moves in the direction of enhancing energy efficiency and user comfort. Smart sensors can monitor various environmental factors like temperature, humidity, occupancy, and air quality in real-time. These data points are then communicated to the HVAC system via IoT technology, allowing it to automatically adjust heating, cooling, and ventilation according to actual needs and preferences. For example, occupancy sensors can detect when a room is empty and reduce heating or cooling accordingly, while weather sensors can adapt the system to external conditions. Integration not only leads to significant energy savings but also offers a personalized and responsive environment. Development of these systems is a move towards more intelligent, connected, and sustainable building management, where HVAC systems are in harmony with occupants' needs and the environment.
These technologies allow real-time monitoring and adjustments, as seen in Singapore's Marina Bay Sands, optimizing energy usage.
Magnetocaloric Cooling:
A new technology in the HVAC industry, it is a significant departure from traditional cooling methods and still in its infancy. The theory does leverage the magnetocaloric effect, where certain materials change temperature when exposed to a magnetic field. By repeatedly applying and removing a magnetic field, the magnetocaloric material can be made to absorb heat from its surroundings and then release it, creating a cooling cycle.
In the context of HVAC systems, Magnetocaloric Cooling offers the potential for highly efficient, environmentally friendly cooling solutions. Unlike conventional refrigeration, which relies on refrigerants that can be harmful to the environment, magnetocaloric systems use solid materials and water-based coolants that are more eco-friendly.
Applications of this technology are still relatively new and limited, but it holds promising potential for energy savings, reduction of greenhouse gas emissions, and the elimination of harmful refrigerants. As research and development continue, Magnetocaloric Cooling could become a cornerstone of sustainable climate control, reshaping how we approach cooling in both residential and commercial settings.
A promising technology, it has only been trialed in various industrial applications.
Challenges and Future Directions
Challenges such as high initial costs, specialized training, and local regulations continue to persist. However, the ongoing push for green buildings, governmental incentives, and research are contributing to overcoming these hurdles.
From geothermal heat pumps to future innovations like magnetocaloric cooling, thermodynamics plays a central role in shaping sustainable HVAC systems. The ongoing application and development of these principles represent a significant contribution to the global green building movement in both heating and air conditioning systems.
Resources:
Moran, M. J., Shapiro, H. N., Boettner, D. D., & Bailey, M. B. (2014). Fundamentals of Engineering Thermodynamics. John Wiley & Sons.
Kutz, M. (2016). Handbook of Environmental Engineering. John Wiley & Sons.
U.S. Department of Energy. (2021). "Energy Saver: Heat Pump Systems." Retrieved from Energy.gov.
"The Bullitt Center" - Bullitt Center Website.
"Geothermal Heating and Cooling at Ball State University" - Ball State University Website.
Additional resources and information on new developments within the industry.
Learn more about our A2L Refrigerant Certification program, as well as our EPA 608 Certification and EPA 609 Certification programs.